This article has been reviewed according to Science X's editorial process and policies. Editors have highlighted the following attributes while ensuring the content's credibility:
fact-checked
trusted source
proofread
Scientists probe the source of stochastic occurrence of super-knock in engines running on hydrogen/methane fuels
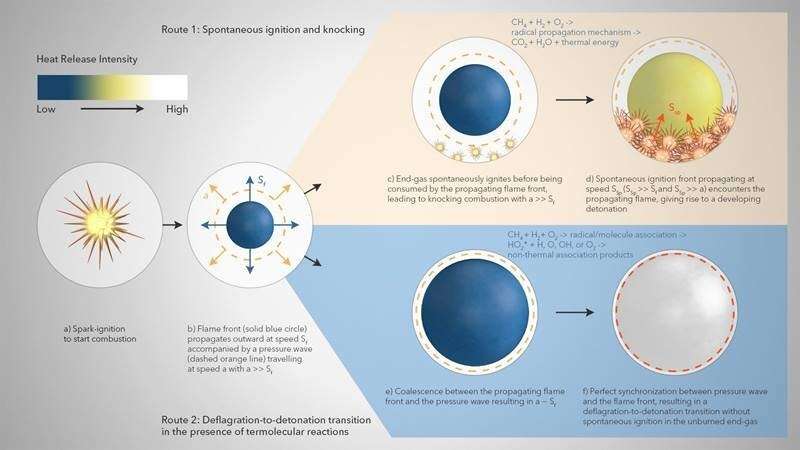
In a study published in Combustion and Flame, researchers investigated the impact of non-thermal reaction chemistry on the propagation of combustion fronts for a H2-CH4 fuel mixture burning in air within a bounded domain, representing an idealized engine cylinder.
Previous work has shown that in some combustion environments, the H + CH3 and H + OH radical-radical recombination and the H + O2 radical-molecule association reactions can form long-lived excited-state intermediates (such as CH4*, H2O*, HO2*) that can undergo subsequent reactions with H, O, OH, and O2 before undergoing collisional stabilization (to CH4, H2O, HO2).
The international team led by Sandia National Laboratories included non-thermal "termolecular" reactions in the model, studying in particular the effects of such radical-radical recombination and radical-molecule association reactions. The team used the S3D direct numerical simulation (DNS) code, with 1 micrometer spatial resolution, showing that inclusion of non-thermalized reaction chemistry influences chemical reaction fluxes during high-pressure H2-CH4 combustion and the transition of deflagration fronts to high-pressure, fast-moving detonation fronts.
On the other hand, the inclusion of chemical explosive mode analysis (CEMA), a reliable computational flame diagnostic tool to systematically detect important species and reactions formed during combustion, indicated that, irrespective of the presence of non-thermal reactivity, temperature and oxygen concentration remain the two most dominant variables affecting detonation formation in H2/CH4-air mixtures under engine relevant conditions.
The researchers note that this particular observation might change with different H2/CH4 blending ratios. The researchers' proposed model appears in the image above. First, a spark-triggered flame propagates outwards at speed Sf accompanied by a pressure wave traveling at speed a, where a is much larger than Sf (a >> Sf). Without termolecular reactions, the unburned gas near the cylinder wall spontaneously ignites before being consumed by the spark-triggered flame.
Subsequently, the emerging ignition front propagates outward with speed 'SSp' such that it remains decoupled from the pressure wave (SSp >> a), thereby leading to the formation of normal knock. However, in the presence of termolecular reactions, coalescence between the spark-triggered flame front and the pressure wave occurs with 'a' being approximately as large as Sf. The perfect synchronization between the pressure wave and the spark-triggered flame front results in the deflagration-to-detonation transition i.e., super-knock without any spontaneous ignition in the unburned end-gas. The researchers propose that their non-thermal reaction chemistry needs to be included when modeling the combustion of H2-CH4 mixtures to accurately predict important aspects of flame behavior.
Small-displacement turbocharged internal combustion engines can experience "knock" without suffering lasting damage. Knock happens when the fuel ignites earlier than expected. Much more damaging is "super-knock." Unlike normal knock, super-knock is caused by a detonation wave due to a feedback loop between the heat release associated with the flame and pressure inside an engine cylinder.
One alternative fuel, hydrogen, is at higher risk of super-knock than other fuels because of the ways engines must operate to run efficiently on hydrogen. One solution is fuels made of a mix of hydrogen and methane. Adding methane to hydrogen fuel can smooth combustion and reduce some types of emissions. Combustion in engines is extremely complex, so researchers need to study hydrogen/methane fuel reactions to aid in engine design.
Using blends of hydrogen and methane as fuels in internal combustion engines is one of the most promising strategies for reducing carbon dioxide emissions. One concern when burning these fuels in spark-ignited engines is the transition from a "desirable" mechanism of combustion to the formation of a detonation wave. In desirable combustion, the wave of fuel combustion (called deflagration) propagates away from the spark ignition source.
Under the wrong conditions, this combustion can instead lead to the formation of a detonation wave. This wave rapidly consumes all the fuel and results in a strong pressure spike called super-knock in engines. To avoid super-knock, scientists studied the causes of the shift from deflagration-to-detonation. This research will help pave the way to the use of viable alternatives to fossil fuels in internal combustion engines.
More information: Swapnil Desai et al, Effects of non-thermal termolecular reactions on detonation development in hydrogen (H2)/methane (CH4)—air mixtures, Combustion and Flame (2022). DOI: 10.1016/j.combustflame.2022.112277
Provided by US Department of Energy